Long Live Radiopharmaceuticals!
Radiotherapy is an oncological mainstay. Targeted radiopharmaceuticals are sweeping the clinical landscape, but face substantial hurdles to realize their maximum potentials.
Introduction
Radioligand therapy (RLT) is one of the most exciting spaces in modern pharma. Anchored in a century of knowledge about radiation and cancer, RLT is a practice-changing therapeutic paradigm. The excitement for RLT flows from its potential to expand into common cancer indications and to earlier lines of treatment. Though the hype seems merited, RLT faces myriad challenges to becoming a standard of care (SOC).
An Abbreviated History
German physicist W. C. Roentgen discovered X-rays in 1895. Three days later, a vacuum tube manufacturer used X-rays to treat a breast cancer patient. By the early 1900’s, physicians understood that radiotherapy could reduce pain for patients with advanced tumors.
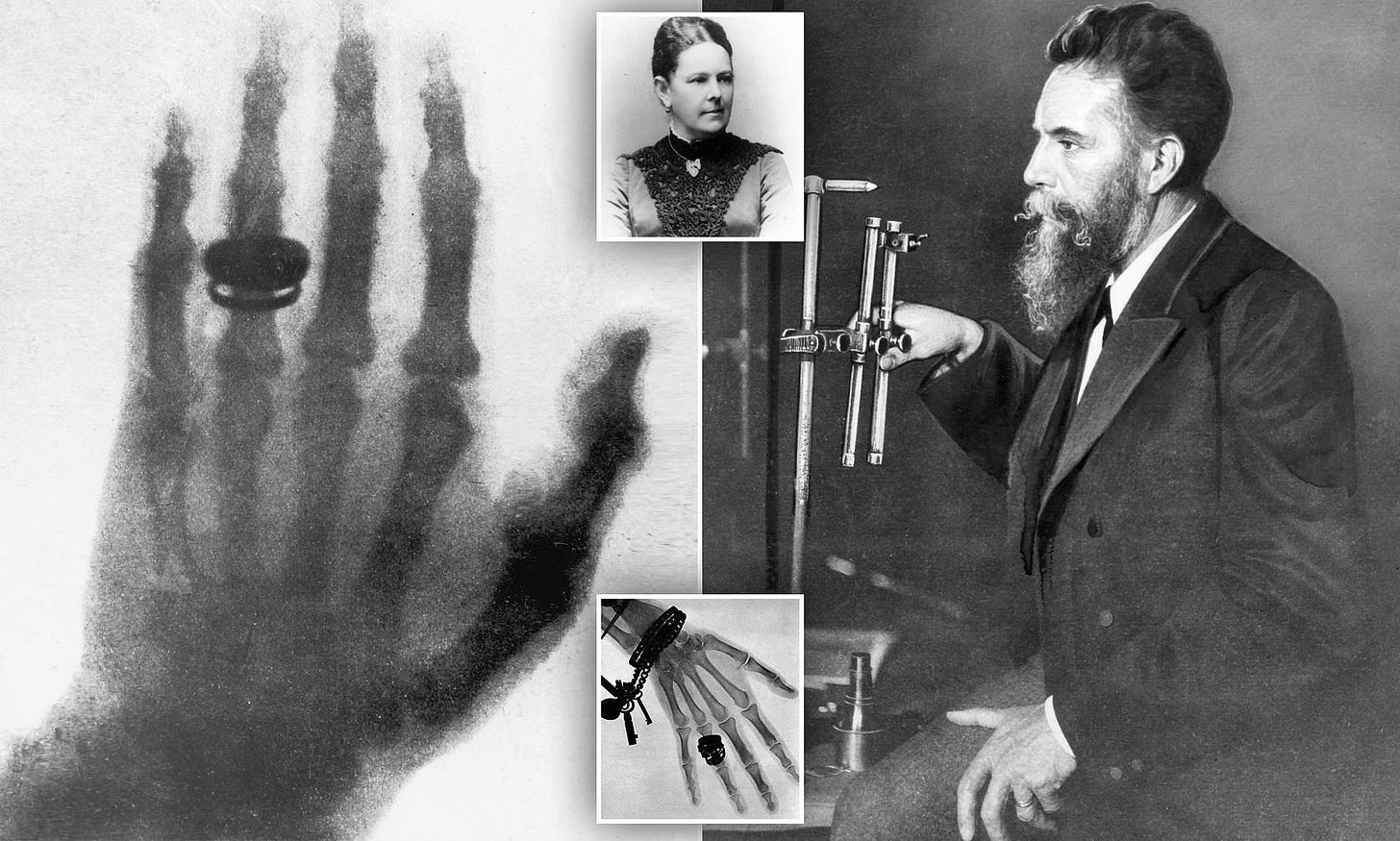
During the 20th century, external beam radiation therapy (EBRT) became an oncological mainstay. Researchers gained a deeper knowledge of radiation’s mechanism of action (MOA). High-energy particles would bombard a cell’s nucleus, lethally shredding its DNA. Quickly dividing cells (e.g., cancer cells) were especially susceptible.
EBRT machines evolved to be more precise and use higher energy radiation. Oncologists developed radiation dosimetry—formulae that helped physicians dial in the exact right amount of radiation. As with other therapies, EBRT had the potential to be toxic. The radiation beam had to travel through normal tissue to reach the tumor after all.
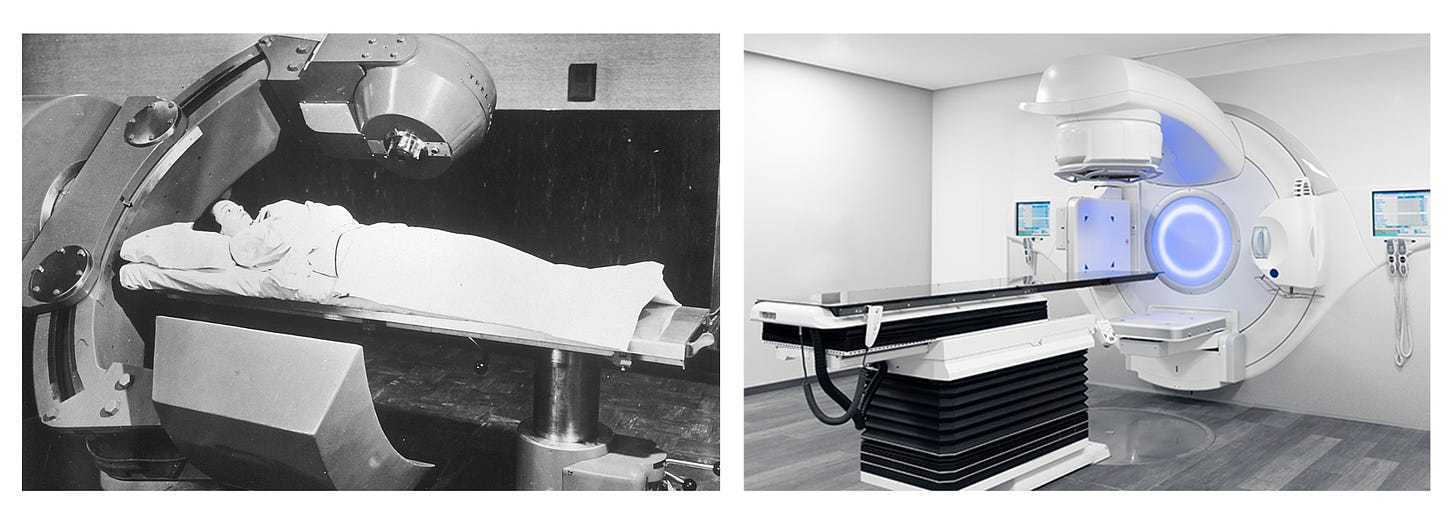
Personalized dosimetry got a boost in the 1980’s when scientists developed peptide receptor scintigraphy (PRS). PRS leveraged the field’s burgeoning knowledge on cell surface proteins. The method involved labeling an antibody with a low-energy, radioactive isotope. Aimed at proteins over-expressed on tumors, the labeled-antibody would accumulate on the surfaces of malignant cells. The label would lit up tumor masses and any metastases, informing dynamic dose calculations.
By the early 1990’s, oncologists extended this concept to treatment. Peptide receptor radionuclide therapy (PRRT) exchanged the low-energy radionuclide for a high-energy one—one powerful enough to kill surrounding cells. Conceptually, PRRT was thought to avoid the toxicities of EBRT by ensuring precision in radiation delivery. PRRT would serve as the genesis of contemporary RLT.
Radioligands 101
“If you can see it, you can treat it.” —Anonymous
Contemporary radioligand therapy is built on the concept of theranostics—the combination of therapeutics and diagnostics to treat disease. RLTs are modular, multipartite medicines. They’re composed of four parts—a targeting agent (e.g., a peptide analogue), a chemical linker, a chelator, and the radioactive payload. By exchanging the high-energy payload for a low-energy one, an RLT can be converted from a therapeutic compound to a diagnostic one to aid in imaging.
Each component of an RLT influences the efficacy and safety of the drug. Pharmaceutical companies must carefully mix, match, and re-engineer each element to fit a certain target product profile (TPP) for whatever indication the group is aiming at. There are excellent reviews on this topic that interested readers should check out. For the sake of this piece, I’ve summarized the essentials:
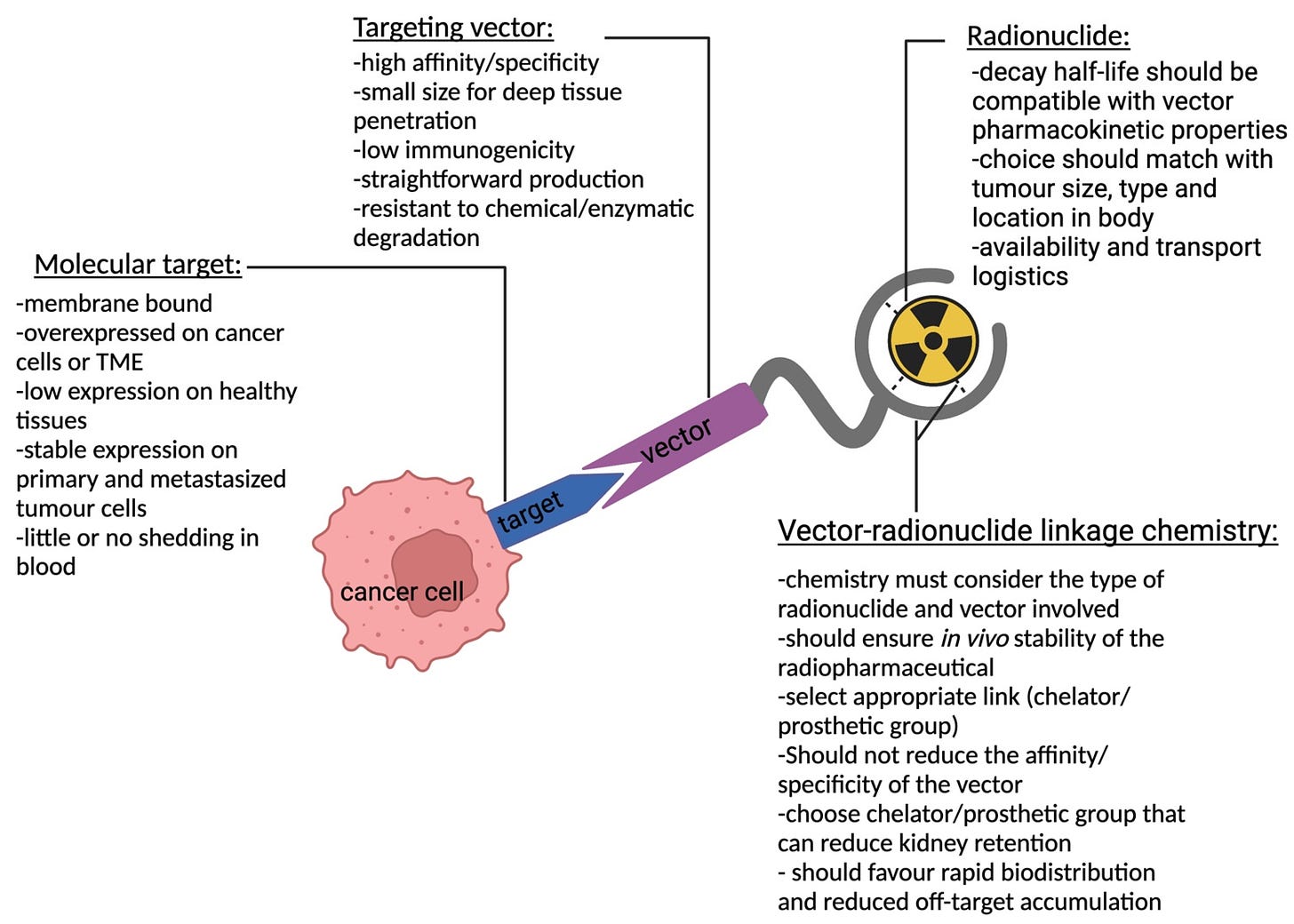
Targeting Agent (Warhead)
Warheads bind to cell surface target proteins. They are the bulk of the mass of any RLT and contribute greatly to its physiochemical properties. Unlike antibody drug conjugates (ADCs), most RLT warheads are smaller vectors, such as small molecules, peptides, or antibody fragments. This is because smaller warheads enable deeper tumor tissue penetration, allowing radiation to affect the interior regions of tumors.
Ideal warheads have high affinity for their targets, increasing the RLT’s residence time and the dose of radiation exerted on a tumor. Warheads should be selective for their targets, minimizing radiation to healthy tissues. Warheads should not instigate a strong immune response and they should be readily manufacturable at scale.
Linkers
Linkers are chemical agents that bind the warhead to the payload group. They should be stable enough to not degrade in plasma, which could cause systemic toxicities. The size, flexibility, and charge distribution of linkers are all relevant design characteristics.
Chelator
Chelators are molecules that form multiple bonds with a central metal atom or ion, acting like a sequestering agent or cage. For RLTs, chelators trap the radionuclide and hold it in place.
Ideal chelators are kinetically inert and thermodynamically stable. Macrocyclic chelators are common as they form a stable ring around a radionuclide. however, the high number of coordinate bonds makes for harder chelator synthesis. Acyclic chelators are easier to produce, but less stable.
Radionuclide
The choice of radionuclide is critical. As shown in the table below, drug designers must choose between an alpha or beta emitter. The former releases large amounts of energy over a short range while the latter releases smaller radiation salvos over long distances. Despite the field’s increasing interest in alpha emitters, my sense is that there’s plenty of room for both emitter types.
The second decision involves the specific isotope, which depends on factors to do with half-life, emission dynamics, decay intensity, decay chain, handling considerations, and other logistics.
Why is Everyone Excited About RLT?
RLTs are caught in the zeitgeist for two reasons—their intrinsic attributes and the strength of incremental clinical data.
Radioligands’ theranostic capability opens up unique doors. Specifically, physicians can verify in vivo biodistribution in a manner not possible with other forms of targeted therapy. This aids personalized dosimetry. RLTs have an essentially guaranteed MOA. As mentioned earlier, these drugs lean on a century of medical knowledge and know-how. Radiation simply works. This fact makes RLTs more attractive than ADCs in some ways since there’s no compounded risk that a novel payload isn’t efficacious. Beyond these intrinsic advantages, the clinical data speaks for itself.
The beachhead indication for RLT is mid-gut neuroendocrine tumors (NETs). Established and emerging RLTs for NETs often target the somatostatin receptor SSTR2. In January 2017, one of the largest Phase III RLT studies (NETTER-1) showed that the drug 177-Lu-DOTA extended both quality and quantity of life for patients with advanced NETs. This trial served as the basis for the 2018 FDA approval of Novartis’s Lutathera for patients with SSTR2 (+) NETs.
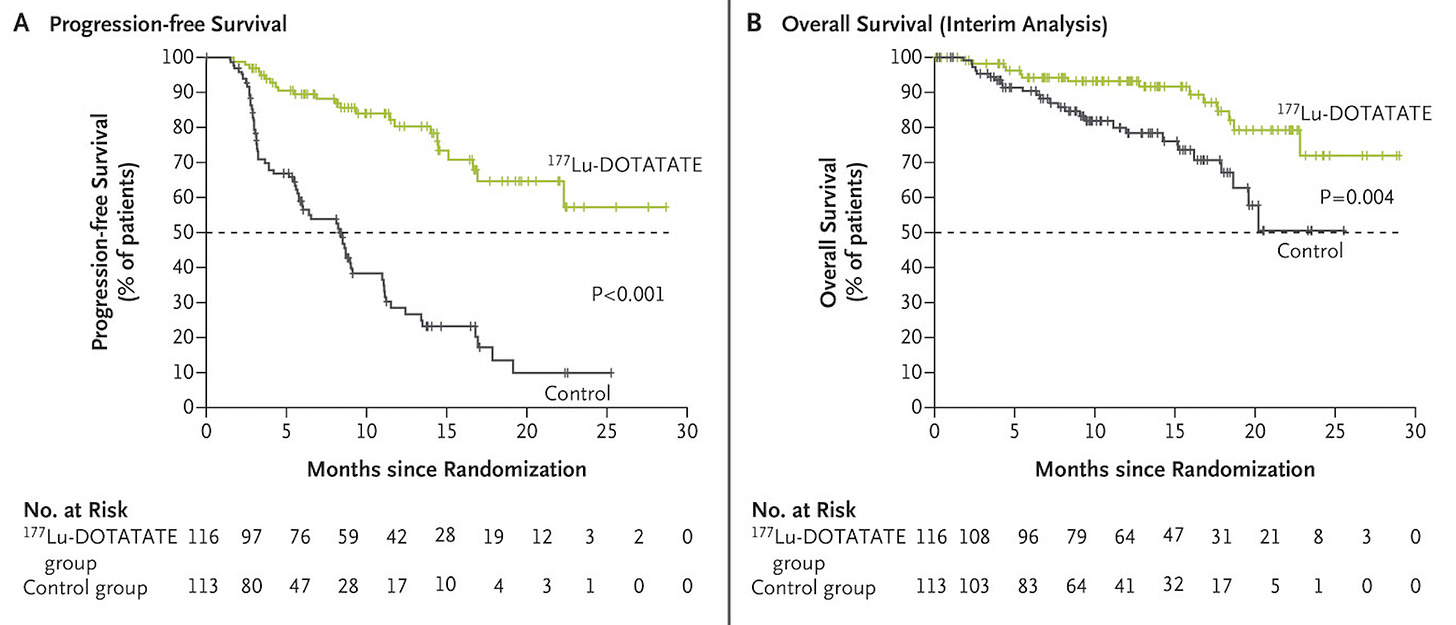
Six months ago, the follow-up NETTER-2 study delivered its first tranche of results. The trial enrolled 226 patients to receive Lutathera or the current standard-of-care (SOC) (octreotide) in the first line (1L) setting for NETs. Compared to the SOC, Lutathera improved median progression free survival (PFS) from 8.5 months to 22.5 months. Importantly, the overall response rate (ORR) expanded nearly five-fold from 9% to 43%. Fewer than five serious adverse events (SAEs) were observed. The expansion from a later line to a 1L treatment speaks to the value and market growing potential of RLTs.
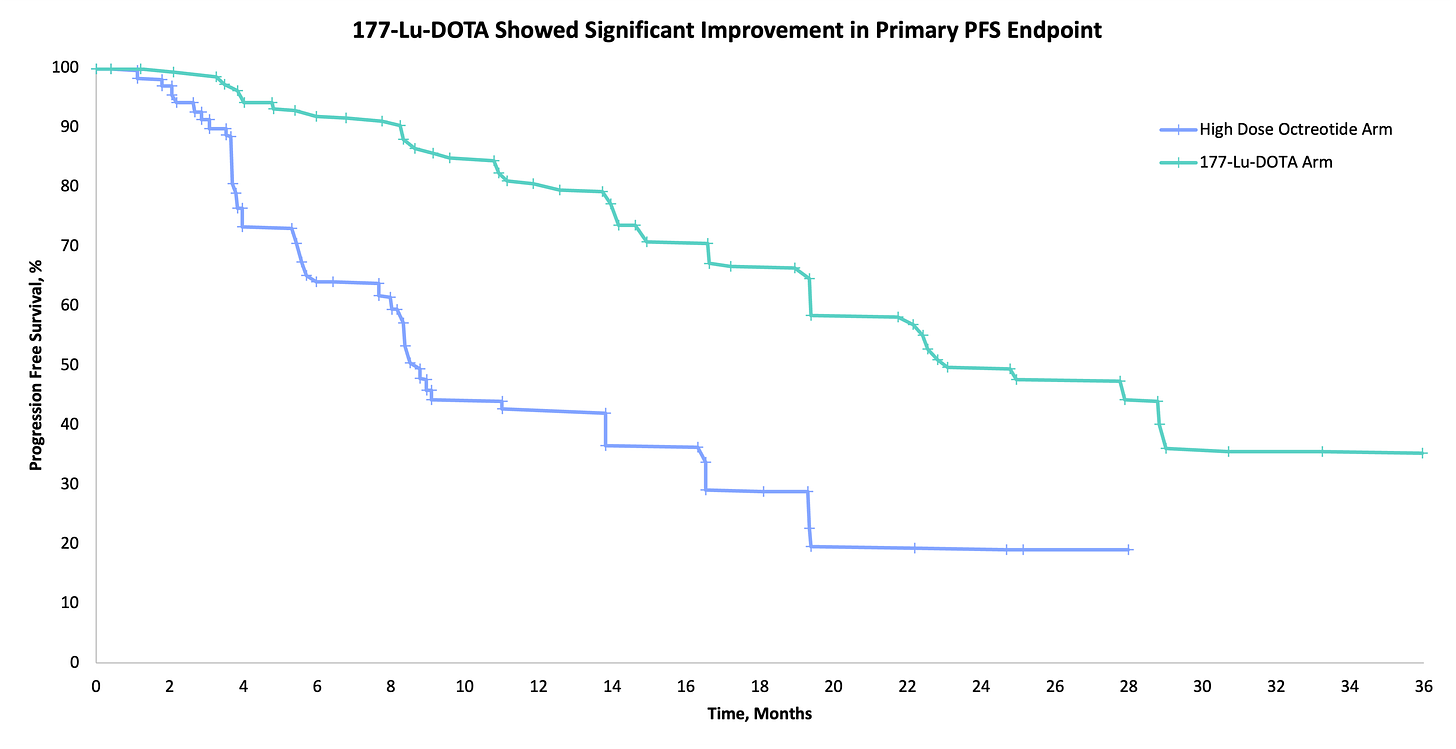
Metastatic, castration-resistant prostate cancer (mCRPC) is the second largest RLT application today. Also approved by the FDA, Novartis’s Pluvicto is a similar molecule to Lutathera, but aimed at PSMA—a target highly expressed in prostate cancer. Based on results form the VISION and TheraP trials, Pluvicto is indicated for use in later-line subgroups of mCRPC patients. In VISION, Pluvicto increased the median PFS from 4.3 to 8.7 months and increased overall survival materially from 11 to 14 months. TheraP confirmed these results.
Subsequent studies have also suggested the Pluvicto is useful in tumor re-challenge scenarios where more than the standard dose regimen is administered after a dosing holiday, suggesting that these can be more durable therapies. One patient has even undergone >20 cycles over 5 years with no DLTs and evidence of response after each re-challenge.
Okay, What’s the Catch?
One of the questions we kick around a lot at Dimension is, “Is the juice worth the squeeze?” Are the challenges with making and scaling RLTs worth the value they bring to patients and investors? We believe so.
The oft-referenced obstacle with RLTs is logistics. Some go so far as to say that RLT logistics are as complex as personalized cell therapies. While this piece isn’t about RLT manufacturing and logistics, these are critical points to bring up.
Manufacturing timelines are ultra-compressed because therapeutic radionuclides decay on the order of days. These isotopes are generally produced in highly-specialized, central facilities with particle accelerators. Raw elements are bombarded with high-energy particles, allowed to cool, and then swiftly packaged in lead containers and sent to vendors for assembly. Nuclides are added to the chelator and then labeled to the vector using a variety of bio-conjugation techniques.
Once assembled and QC’d, the drugs must get to patients usually within 14 days. Anyone who handles or administers RLTs must be an authorized user (AU) as stated by the National Regulatory Commission (NRC). After dosing , radioactive waste must be kept at the site in a specialized storage container for a minimum of 120 days or until the material has no detectable radiation.
Despite all these logistical hurdles, I believe the biggest obstacle for RLTs generally is kidney (renal) toxicity. If this isn’t solved, ameliorating all the logistical challenges becomes moot. Expanding the effectiveness of RLTs requires expanding their therapeutic indexes (TIs).
Nephrotoxicity
Drugs are one of the most common sources of acute kidney damage, causing roughly 20% of renal failure cases.
Drug-induced nephrotoxicity is especially common in older adults. Unfortunately, this demographic also has the highest cancer incidence rate. This poses significant challenges for cancer therapy, which may need to be stopped if renal damage accumulates.
Drugs exert their toxic effects on renal tissue through one or more common pathogenic mechanisms. The first is altering intraglomerular hemodynamics. In healthy young adults, the kidney’s filtration machinery (the glomerulus) processes roughly 120 mL of plasma per minute. Some drugs interrupt the kidney’s ability to regulate glomerular pressure, disrupting the flow of fluid through the kidney.
The second is tubule cell toxicity. Renal tubular cells are especially susceptible to damage since they concentrate and reabsorb filtrate, exposing them to high levels of toxin. A third mechanism is inflammation, which can lead to fibrosis and scaring throughout multiple tissues in the kidney. The list goes on, but these three modes of renal injury are most common, especially for RLTs.
How do RLTs Cause Renal Insult?
RLTs have a renal-predominant mode of excretion. These compounds’ pharmacokinetic (PK) properties cause kidney retention. Taken together, these facts explain why renal dose-limiting toxicities (DLTs) may narrow the therapeutic indexes (TIs) of RLTs. Oncologists may not be able to administer clinically meaningful RLT doses before signs of renal injury manifest.
Using the aforementioned nephrotoxicity framework, RLTs primarily cause tubule cell toxicity. Recall that most RLTs use small binders to increase tumor tissue penetration. Compounds below the glomular filtration barrier (60 kDa) are processed in the main, functional units of they kidney called nephrons. These are dense networks of tubes that allow biomolecular interchange between blood and urine, allowing the body to reabsorb things it wants while excreting things it doesn’t.
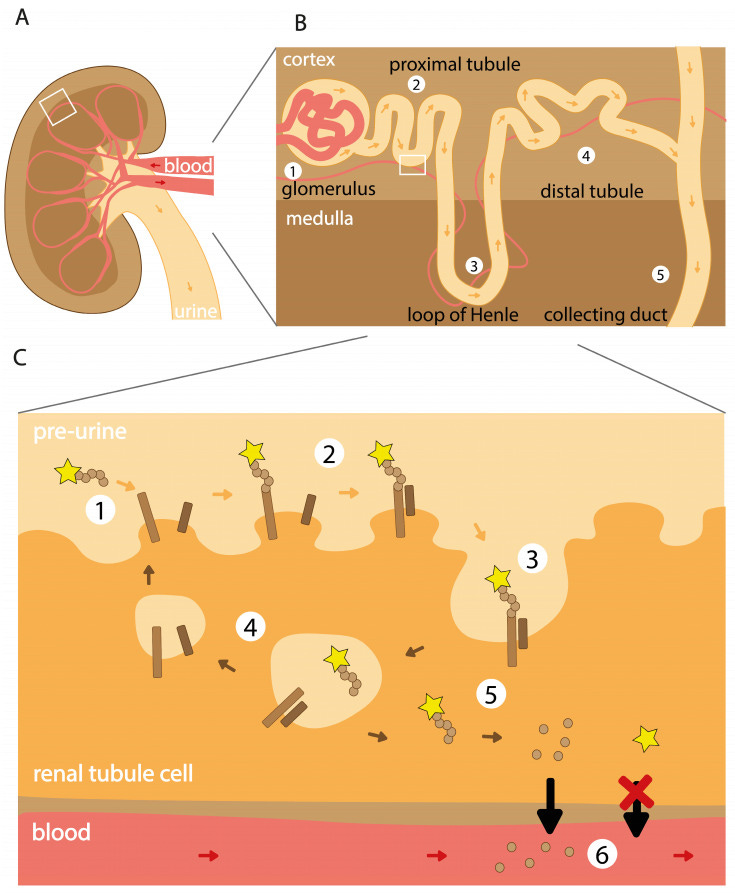
As shown above, renal tubule cells have evolved a network of surface receptors to detect and facilitate the reabsorption of critical-to-life biomolecules. Megalin and cubilin are two such receptors that are relevant to RLT toxicity. Because most RLT targeting vectors are based on natural human biomolecules, they’re highly susceptible to uptake by megalin and cubilin.
Once internalized, RLTs are metabolized inside lysosomes. While peptide fragments may find their way back into the bloodstream, nuclides generally dwell inside tubule cells—allowing toxic radiation to accumulate, causing collateral DSBs and oxidative stress.
Other RLT Parameters Affecting Nephrotoxicity
Beyond molecular weight (MW), the primary determinants of renal toxicity include RLT charge and charge distribution, nuclide residualizing activity, chelator stability, and target selection.
The surfaces of proximal tubule cells are generally negatively charged. By adding more negatively charged groups to the linker or warhead, it creates a less favorable surface interaction for renal uptake. However, the charge distribution matters too. Positively charged local regions, like chelator-nuclide groups, can interact with megalin/cubilin and undergo active transport into tubule cells.
Certain radionuclides cause greater kidney damage than others. For example, radiohalogens cause less nephrotoxicity than radiometals. Radiohalogens get metabolized into smaller metabolites and are more easily transported back into blood whereas radiometals can form larger byproducts, causing them to accumulate in tubule cells. Unfortunately, almost all therapeutic nuclides are metals. This is known as residualizing activity.
Beyond the nuclide, the chelation shell’s stability affects toxicity since free radioisotopes can be more damaging than their sequestered counterparts. Should enzymes within the lysosome break the chelation cage, the nuclide would be free to cause more toxicity. This can be doubly challenging for alpha emitters. Alpha emitters frequently recoil like a gun out of their chelation cages, freeing daughter nuclei to continue their decay chain haphazardly.
Target selection plays a pivotal role in kidney toxicity. For example, both SSTR2 and PSMA are expressed in low levels in renal cells. When RLTs bind their cognate targets expressed in the kidney, it constitutes on-target, off-site toxicity.
Renoprotection
Battle-hardened in the clinic, there are several first-generation workarounds to protecting renal cells from RLT-induced injury.
Perhaps the most pressing challenge to solve in the RLT space is that of nephrotoxicity. For decades, clinicians and drug developers have leveraged first-generation renoprotective strategies to mitigate renal injury and expand the TI of RLT drugs. This section summarizes these methods and hints at a more elegant future for renoprotection.
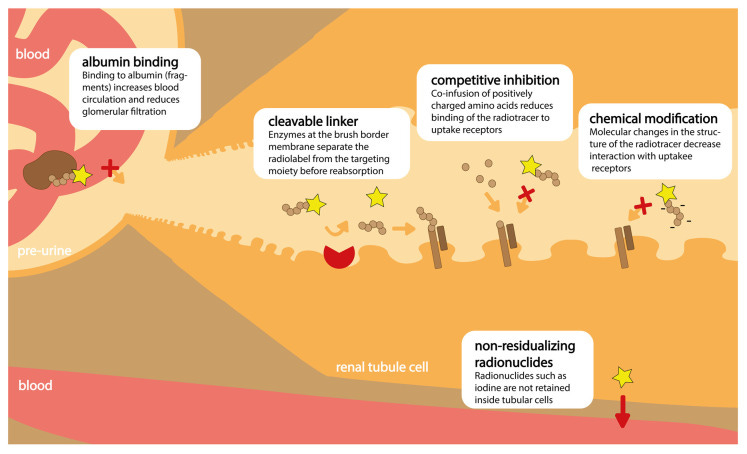
A common technique since the early days of RLT has been to infuse patients with a cocktail of positively charged amino acids (e.g., lysine and arginine). These acids compete with RLTs to bind megalin and cubilin. By occupying receptors with an inert substance, fewer copies of megalin and cubilin are free to bind and internalize potentially damaging radiation. This technique is called competitive inhibition.
As shown below, pre-targeting is another mechanism for sparing renal tissue. This method involves infusing an unlabeled RLT vector which binds to both the tumor and renal cells. A second injection containing the therapeutic nuclide is given. The nuclide binds to the unlabeled vector in vivo, enabling delivery of radiation to target tissue.
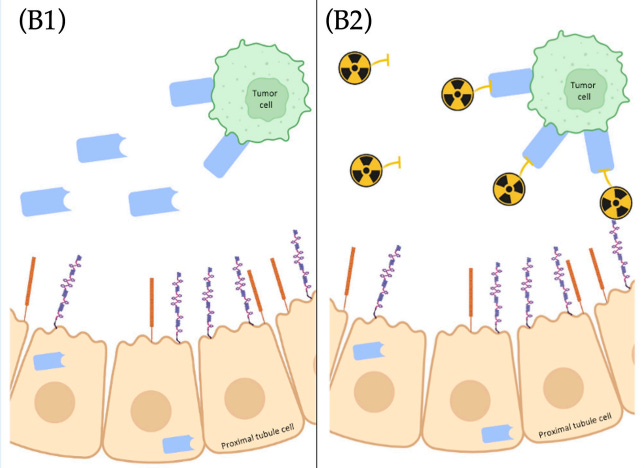
Drug designers have begun installing an albumin-binding moiety onto therapeutic RLTs. Albumin is a long-lived, heavy serum protein. By hitching a ride on the exterior of albumin, RLT residence time in plasma increases. Importantly, the complex is heavy enough to avoid becoming part of the glomular filtrate, lessening the likelihood of renal cell accumulation.
Additionally, designers have leveraged conditionally-cleavable linkers. These species are meant to break apart in the presence of enzymes located on the exteriors of proximal renal tubule cells. Conceptually, by cleaving here, radioactive nuclides are disallowed from accumulating inside kidney cells.
Perhaps one of the most valid, but challenging, techniques for avoiding nephrotoxicity is tuning the physiochemical properties of the RLT itself. As mentioned previously, weight, charge, charge distribution, and more all leave their mark on toxicity.
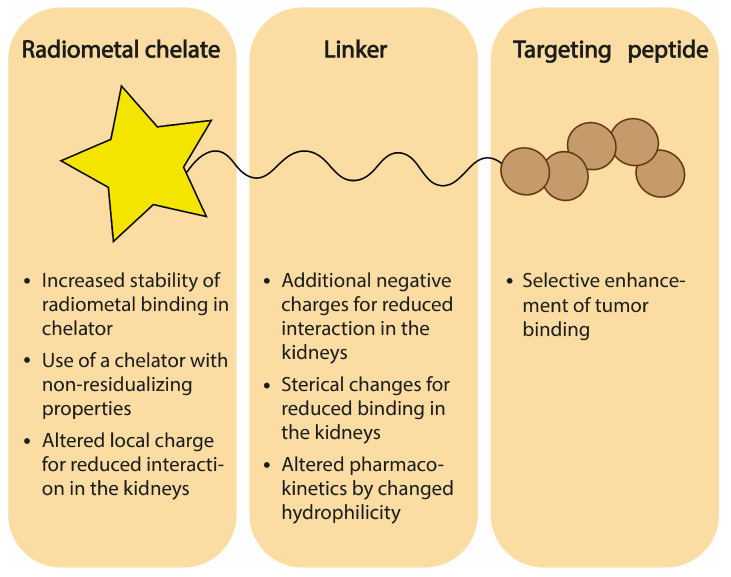
De-Targeting and Other Emerging RLT Trends
It’s the end of the beginning for RLTs. Radiation works. RLTs work. There are still some kinks in the system to work out, but the future looks bright.
Rationally engineering renoprotective properties into RLTs is one of the most critical research thrusts in this space, but it’s not the only one. As the number of RLTs in the clinic grows, it’s clear the field is increasingly adopting alpha radiation, broadening its vector and target choices, and rethinking how to approach logistics challenges. This section summarizes some of these advances.
Engineering De-Targeting
The entire RLT field would benefit from the discovery of general rules for sparing renal tubule cells from injury. First-generation renoprotective strategies have proven somewhat successful, but are crude. One path forward might be to treat RLT renal insult as a predictive toxicology problem.
Machine learning (ML) excels at domains currently dominated by human heuristics. This is only the cause with sufficiently large and diverse datasets, however. That’s a place where RLT struggles. Though the field has been around for decades, I’m skeptical that existing data repositories are sufficient for ML model training. Here are the main issues in my mind:
The majority of clinical data is concentrated on similar target-compound pairs, including SSTR2 and PSMA. This could affect a model’s ability to generalize to other modalities (e.g., antibody fragments) or anywhere far outside this distribution.
Though imaging data shows in vivo biodistribution, a unique feature of theranostics, the resolution may not be fine enough to assess whether injury to renal tubule cells is occurring.
There are simply not that many unique agents that’ve been tested in the clinical domain, certainly not enough for ML.
This suggests an enormous amount of highly curated data needs to be constructed first. I’m still on the fence about this, but I’m intrigued by the idea of high-throughput in vitro imaging techniques coupled to in vivo biodistribution data in an active learning format. There are holes here too, but it’s something I’m thinking about.
Evolving Nuclides
Highlighted below is a breakdown of the split between alpha and beta emitters featured in RLTs across various phases of development. Newer drugs are clearly leaning towards including alpha emitters. Alpha emitters are much more likely to deliver cell-killing levels of radiation owing to their relatively higher emission strengths. In theory, this should enable efficacy in scenarios where only a few copies of the drug are delivered to a cell. I do think beta emitters have a significant role still in sufficiently advanced, heterogeneous tumors. With their longer ranges, beta emitters can kill cells that don’t express the target antigen—a common scenario in tumors with multiple, dominant clones.
Manufacturing and Logistics
It’s no surprise that virtually all of the large acquisitions in the RLT space were of companies with verticalized manufacturing. Even so, there’s still some innovation in the space worth mentioning. Companies like ARTBIO and AdvanCell have switched to lead (Pb) isotopes. Unlike other emitters, lead can be manufactured in a bench top format, ostensibly allowing these companies to decouple from centralized production facilities. Meanwhile, groups like Nusano are leveraging novel technology to shore up our isotope supply. Nusano operates a linear particle beam technology they claim can simultaneously generate multiple therapeutically relevant isotopes.
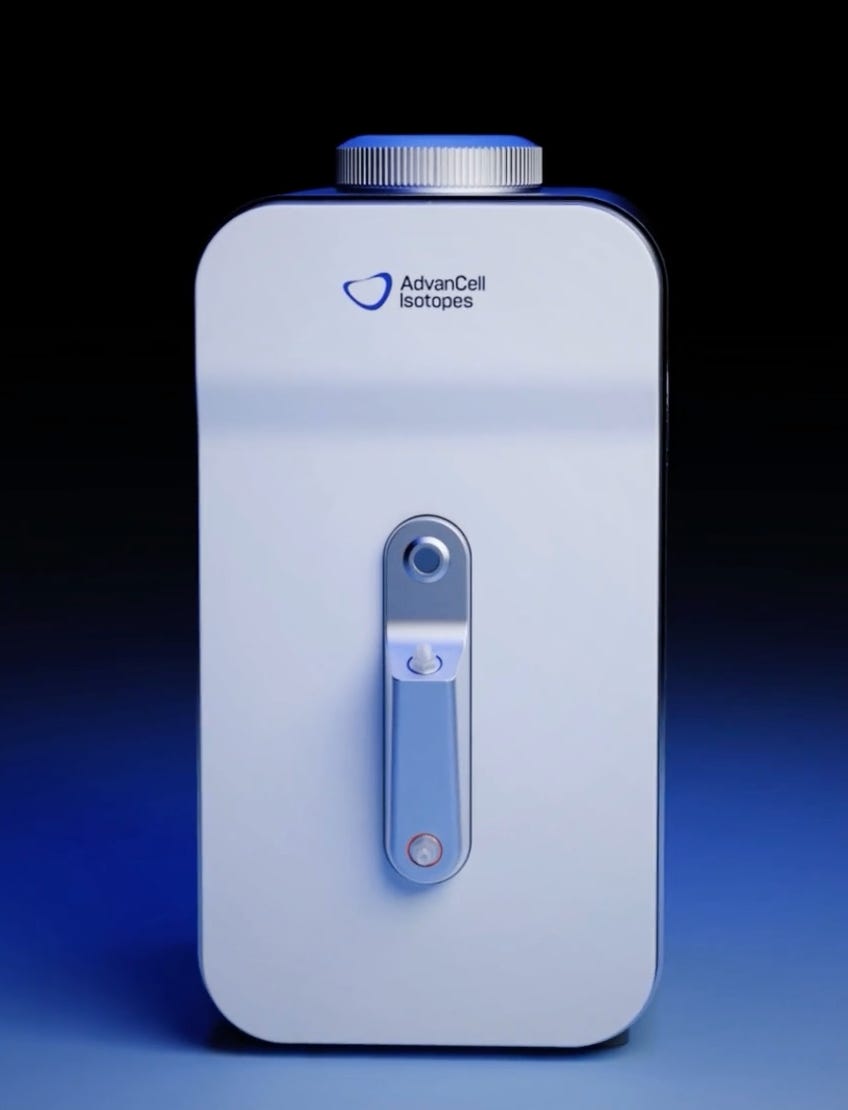
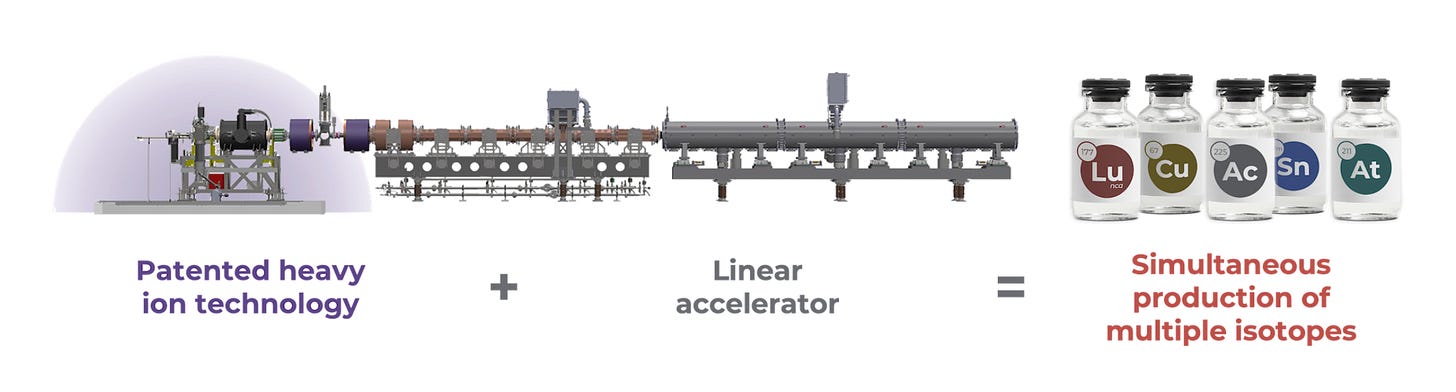
Parting Thoughts
We’ve got a lot of conviction in RLTs. There’s still some ambiguity around increased survival from recent clinical readouts in mCRPC, but we’re optimistic they’ll pan out. There’s been enough investment in the space to really crack open the opportunity in other indication areas. Unlike other modalities, we know RLT’s MOA works. It’s pesky nephrotoxicity, clarity around nuclide and isotope choice, and logistics that are gating farther progress. All of these are areas we’re actively investigating. If you have strong opinions or are working in the space—send me a DM!